The purpose of the project is to provide a comprehensive theoretical description of simultaneous coherent interaction of optical and mechanical (acoustic) fields with quantum emitters in solids. Based on this fundamental understanding, we will propose and describe methods to exchange and convert quantum information between physically dissimilar degrees of freedom (charge, spin, light and sound) within a hybrid solid-state system. In this way, we will work out a theoretical framework for controlling the acoustic degrees of freedom at the quantum level, reading out their state, as well as using them as a quantum bus linking qubits or connecting qubits to communication channels. This work is motivated by the idea of a hybrid quantum system that combine complementary strengths of dissimilar physical subsystems, while at the same time avoiding their individual shortcomings. Here, we will work towards reciprocal transduction of quantum information between mechanical (acoustic) and optical degrees of freedom in a solid state system.
We will study solid-state optical quantum emitters, including self-assembled quantum dots (QDs) and defect centers (DCs) in diamond that are coupled to vibrational degrees of freedom of the crystal. For the mechanical degrees of freedom we choose to study the promising and technologically viable surface acoustic waves and confined waves in slabs and membranes (i.e., Rayleigh and Lamb waves). The models will range from single quantum emitters modulated by classical acoustic waves to systems coupled to quantized acoustic waves in acoustic resonators.
The three main areas of the proposed research are: (1) Modeling acoustic cavities and couplings between optical emitters and strain; here we will model strain fields in phononic resonators and waveguides, describe strain coupling to solid-state quantum emitters in such structures, investigate the nonlinear dynamics of the technologically promising heterointegrated systems with LiNbO3 resonators, and model the losses of the resonators to assess their quality. (2) Semiclassical dynamics and relaxation of quantum emitters coupled to acoustic waves and confined acoustic modes; we will study the resonance fluorescence spectra of emitters modulated by acoustic waves and environmental random noise, describe coherence properties of light scattered on acoustically modulated QDs, propose schemes for acousto-optic control of QD spin states, and describe relaxation in QD and DC systems coupled to engineered phononic reservoirs. (3) Quantum dynamics of quantum emitters coupled to mechanical modes; we will analyze coupling between quantum systems mediated by phonon modes, study the quantum effects of mechanical nonlinearity both inherent and induced by coupling to a two-level system, develop single-shot readout protocol for phonon states via statistics of light scattering on the single-photon level, study the transfer of quantum state and entanglement from acoustic states to light, and investigate the effects of optical interactions with the quantum emitter on the quantum state of the coupled mechanical mode to determine schemes of quantum information transfer from optical to phonon modes.
In our work we will use a combination of methods from quantum optics, theory of quantum open systems, many-particle physics and solid state physics. Coupling of quantum emitters to strain fields will be modeled using k.p theory as well as group theory and method of invariants. Dynamical simulations involving dissipation will be performed within the density matrix formalism either by employing Master equations and stochastic techniques of quantum jumps, or by approximate methods like correlation expansions. Wherever possible, the exact description based on the generating functions technique will be used. In addition, finite-element methods will be used for modeling the properties of confined acoustic modes in resonators and their coupling to quantum emitters, along with analytical methods of solid-state acoustics for simple geometries.
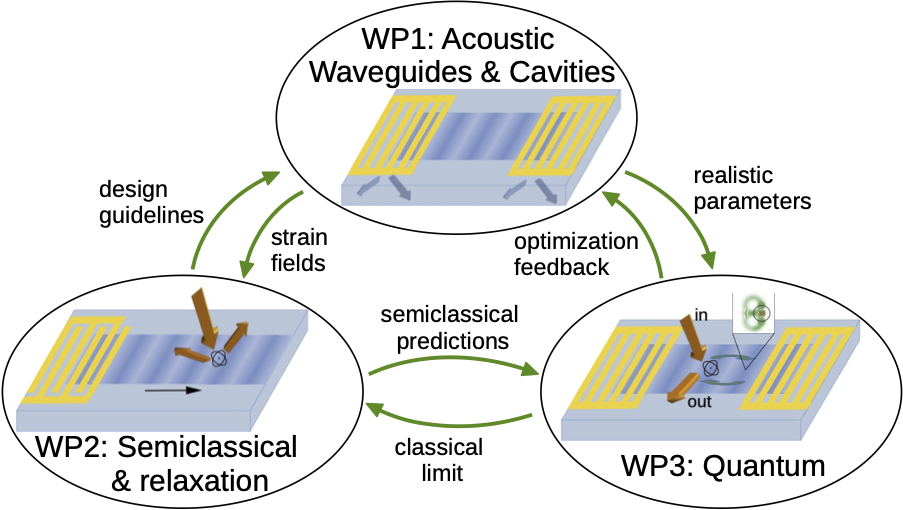